James Webb Space Telescope facts for kids
![]() Rendering of the fully deployed James Webb Space Telescope
|
|
Names | Next Generation Space Telescope (NGST; 1996–2002) |
---|---|
Mission type | Astronomy |
Operator | STScI (NASA) / ESA / CSA |
Website | |
Mission duration |
|
Spacecraft properties | |
Manufacturer |
|
Launch mass | 6,500 kg (14,300 lb) |
Dimensions | 21.197 m × 14.162 m (69.54 ft × 46.46 ft), sunshield |
Power | 2 kW |
Start of mission | |
Launch date | 25 December 2021UTC | , 12:20
Rocket | Ariane 5 ECA+ (S/N 5113, Flight VA256) |
Launch site | Guiana, ELA-3 |
Contractor | Arianespace |
Entered service | 12 July 2022 |
Orbital parameters | |
Reference system | Sun–Earth L2 orbit |
Regime | Halo orbit |
Periapsis | 250,000 km (160,000 mi) |
Apoapsis | 832,000 km (517,000 mi) |
Period | 6 months |
Main telescope | |
Type | Korsch telescope |
Diameter | 6.5 m (21 ft) |
Focal length | 131.4 m (431 ft) |
Focal ratio | f/20.2 |
Collecting area | 25.4 m2 (273 sq ft) |
Wavelengths | 0.6–28.5 μm (orange to mid-infrared) |
![]() James Webb Space Telescope mission logo Large Strategic Science Missions
Astrophysics Division |
The James Webb Space Telescope (JWST) is a space telescope designed to conduct infrared astronomy. As the largest telescope in space, it is equipped with high-resolution and high-sensitivity instruments, allowing it to view objects too old, distant, or faint for the Hubble Space Telescope. This enables investigations across many fields of astronomy and cosmology, such as observation of the first stars and the formation of the first galaxies, and detailed atmospheric characterization of potentially habitable exoplanets.
Although the Webb's mirror diameter is 2.7 times larger than that of the Hubble Space Telescope, it produces images of comparable resolution because it observes in the longer-wavelength infrared spectrum. The longer the wavelength of the spectrum, the larger the information-gathering surface required (mirrors in the infrared spectrum or antenna area in the millimeter and radio ranges) for an image comparable in clarity to the visible spectrum of the Hubble Space Telescope.
The Webb was launched on 25 December 2021 on an Ariane 5 rocket from Kourou, French Guiana. In January 2022 it arrived at its destination, a solar orbit near the Sun–Earth L2 Lagrange point, about 1.5 million kilometers (930,000 mi) from Earth. The telescope's first image was released to the public on 11 July 2022.
The U.S. National Aeronautics and Space Administration (NASA) led Webb's design and development and partnered with two main agencies: the European Space Agency (ESA) and the Canadian Space Agency (CSA). The NASA Goddard Space Flight Center in Maryland managed telescope development, while the Space Telescope Science Institute in Baltimore on the Homewood Campus of Johns Hopkins University operates Webb. The primary contractor for the project was Northrop Grumman.
The telescope is named after James E. Webb, who was the administrator of NASA from 1961 to 1968 during the Mercury, Gemini, and Apollo programs.
Webb's primary mirror consists of 18 hexagonal mirror segments made of gold-plated beryllium, which together create a 6.5-meter-diameter (21 ft) mirror, compared with Hubble's 2.4 m (7 ft 10 in). This gives Webb a light-collecting area of about 25 m2 (270 sq ft), about six times that of Hubble. Unlike Hubble, which observes in the near ultraviolet and visible (0.1 to 0.8 μm), and near infrared (0.8–2.5 μm) spectra, Webb observes a lower frequency range, from long-wavelength visible light (red) through mid-infrared (0.6–28.5 μm). The telescope must be kept extremely cold, below 50 K (−223 °C; −370 °F), so that the infrared light emitted by the telescope itself does not interfere with the collected light. Its five-layer sunshield protects it from warming by the Sun, Earth, and Moon.
Initial designs for the telescope, then named the Next Generation Space Telescope, began in 1996. Two concept studies were commissioned in 1999, for a potential launch in 2007 and a US$1 billion budget. The program was plagued with enormous cost overruns and delays. A major redesign was accomplished in 2005, with construction completed in 2016, followed by years of exhaustive testing, at a total cost of US$10 billion.
Contents
Features
The James Webb Space Telescope has a mass that is about half of Hubble Space Telescope's mass. The JWST has a 6.5-meter (21 ft)-diameter gold-coated beryllium primary mirror made up of 18 separate hexagonal mirrors. The mirror has a polished area of 26.3 m2 (283 sq ft), of which 0.9 m2 (9.7 sq ft) is obscured by the secondary support struts, giving a total collecting area of 25.4 m2 (273 sq ft). This is over six times larger than the collecting area of Hubble's 2.4-meter (7.9 ft) diameter mirror, which has a collecting area of 4.0 m2 (43 sq ft). The mirror has a gold coating to provide infrared reflectivity and this is covered by a thin layer of glass for durability.
JWST is designed primarily for near-infrared astronomy, but can also see orange and red visible light, as well as the mid-infrared region, depending on the instrument. It can detect objects up to 100 times fainter than Hubble can, and objects much earlier in the history of the universe, back to redshift z≈20 (about 180 million years cosmic time after the Big Bang). For comparison, the earliest stars are thought to have formed between z≈30 and z≈20 (100–180 million years cosmic time), and the first galaxies may have formed around redshift z≈15 (about 270 million years cosmic time). Hubble is unable to see further back than very early reionization at about z≈11.1 (galaxy GN-z11, 400 million years cosmic time).
The design emphasizes the near to mid-infrared for several reasons:
- high-redshift (very early and distant) objects have their visible emissions shifted into the infrared, and therefore their light can only be observed today via infrared astronomy;
- infrared light passes more easily through dust clouds than visible light
- colder objects such as debris disks and planets emit most strongly in the infrared;
- these infrared bands are difficult to study from the ground or by existing space telescopes such as Hubble.
Ground-based telescopes must look through Earth's atmosphere, which is opaque in many infrared bands (see figure of atmospheric absorption). Even where the atmosphere is transparent, many of the target chemical compounds, such as water, carbon dioxide, and methane, also exist in the Earth's atmosphere, vastly complicating analysis. Existing space telescopes such as Hubble cannot study these bands since their mirrors are insufficiently cool (the Hubble mirror is maintained at about 15 °C [288 K; 59 °F]) thus the telescope itself radiates strongly in the infrared bands.
JWST can also observe nearby objects, including objects in the Solar System, having an apparent angular rate of motion of 0.030 arc seconds per second or less. This includes all planets and satellites, comets, and asteroids beyond Earth's orbit, and "virtually all" known Kuiper Belt Objects. In addition, it can observe opportunistic and unplanned targets within 48 hours of a decision to do so, such as supernovae and gamma ray bursts.
Orbit
The JWST is in orbit far from Earth, to avoid heat radiating from the Earth and moon. This special orbit is beyond the moon, at the second Lagrange point (L2) of the Sun-Earth system, a place of stable gravity. This orbit is 1,500,000 kilometres (930,000 miles) from Earth, about four times farther away from us than the moon. This keeps it in the Earth's shadow most of the time; it does not actually go around the Earth, but goes around the sun at the same speed as the Earth.
Mission goals
The James Webb Space Telescope has four key goals:
- to search for light from the first stars and galaxies that formed in the universe after the Big Bang
- to study galaxy formation and evolution
- to understand star formation and planet formation
- to study planetary systems and the origins of life
These goals can be accomplished more effectively by observation in near-infrared light rather than light in the visible part of the spectrum. For this reason, JWST's instruments will not measure visible or ultraviolet light like the Hubble Telescope, but will have a much greater capacity to perform infrared astronomy. JWST will be sensitive to a range of wavelengths from 0.6 to 28 μm (corresponding respectively to orange light and deep infrared radiation at about 100 K or −173 °C).
JWST may be used to gather information on the dimming light of star KIC 8462852, which was discovered in 2015, and has some abnormal light-curve properties.
Additionally, it will be able to tell if an exoplanet has methane in its atmosphere, allowing astronomers to determine whether or not the methane is a biosignature.
Ground support and operations
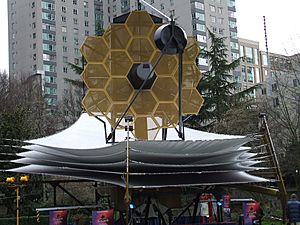
The Space Telescope Science Institute (STScI), in Baltimore, Maryland, on the Homewood Campus of Johns Hopkins University, was selected as the Science and Operations Center (S&OC) for JWST with an initial budget of US$162.2 million intended to support operations through the first year after launch. In this capacity, STScI will be responsible for the scientific operation of the telescope and delivery of data products to the astronomical community. Data will be transmitted from JWST to the ground via the NASA Deep Space Network, processed and calibrated at STScI, and then distributed online to astronomers worldwide. Similar to how Hubble is operated, anyone, anywhere in the world, will be allowed to submit proposals for observations. Each year several committees of astronomers will peer review the submitted proposals to select the projects to observe in the coming year. The authors of the chosen proposals will typically have one year of private access to the new observations, after which the data will become publicly available for download by anyone from the online archive at STScI.
The bandwidth and digital throughput of the satellite is designed to operate at 458 gigabits of data per day for the length of the mission (equivalent to a sustained rate of 5.42 megabits per second [Mbps]). Most of the data processing on the telescope is done by conventional single-board computers. The digitization of the analog data from the instruments is performed by the custom SIDECAR ASIC (System for Image Digitization, Enhancement, Control And Retrieval Application Specific Integrated Circuit). NASA stated that the SIDECAR ASIC will include all the functions of a 9.1 kg (20 lb) instrument box in a 3 cm (1.2 in) package and consume only 11 milliwatts of power. Since this conversion must be done close to the detectors, on the cold side of the telescope, the low power dissipation is crucial for maintaining the low temperature required for optimal operation of JWST.
Comparison with other telescopes
The desire for a large infrared space telescope traces back decades. In the United States, the Space Infrared Telescope Facility (SIRTF, later called the Spitzer Space Telescope) was planned while the Space Shuttle was in development, and the potential for infrared astronomy was acknowledged at that time. Unlike ground telescopes, space observatories were free from atmospheric absorption of infrared light. Space observatories opened up a whole "new sky" for astronomers.
The tenuous atmosphere above the 400 km nominal flight altitude has no measurable absorption so that detectors operating at all wavelengths from 5 μm to 1000 μm can achieve high radiometric sensitivity.
However, infrared telescopes have a disadvantage: they need to stay extremely cold, and the longer the wavelength of infrared, the colder they need to be. If not, the background heat of the device itself overwhelms the detectors, making it effectively blind. This can be overcome by careful spacecraft design, in particular by placing the telescope in a dewar with an extremely cold substance, such as liquid helium. The coolant will slowly vaporize, limiting the lifetime of the instrument from as short as a few months to a few years at most.
In some cases, it is possible to maintain a temperature low enough through the design of the spacecraft to enable near-infrared observations without a supply of coolant, such as the extended missions of Spitzer Space Telescope and Wide-field Infrared Survey Explorer, which operated at reduced capacity after coolant depletion. Another example is Hubble's Near Infrared Camera and Multi-Object Spectrometer (NICMOS) instrument, which started out using a block of nitrogen ice that depleted after a couple of years, but was then replaced during the STS-109 servicing mission with a cryocooler that worked continuously. The James Webb Space Telescope is designed to cool itself without a dewar, using a combination of sunshields and radiators, with the mid-infrared instrument using an additional cryocooler.
Name | Launch Year | Wavelength (μm) |
Aperture (m) |
Cooling |
---|---|---|---|---|
Spacelab Infrared Telescope (IRT) | 1985 | 1.7–118 | 0.15 | Helium |
Infrared Space Observatory (ISO) | 1995 | 2.5–240 | 0.60 | Helium |
Hubble Space Telescope Imaging Spectrograph (STIS) | 1997 | 0.115–1.03 | 2.4 | Passive |
Hubble Near Infrared Camera and Multi-Object Spectrometer (NICMOS) | 1997 | 0.8–2.4 | 2.4 | Nitrogen, later cryocooler |
Spitzer Space Telescope | 2003 | 3–180 | 0.85 | Helium |
Hubble Wide Field Camera 3 (WFC3) | 2009 | 0.2–1.7 | 2.4 | Passive, and thermo-electric |
Herschel Space Observatory | 2009 | 55–672 | 3.5 | Helium |
James Webb Space Telescope | 2021 | 0.6–28.5 | 6.5 | Passive, and cryocooler (MIRI) |
JWST's delays and cost increases can be compared to those of its predecessor, the Hubble Space Telescope. When Hubble formally started in 1972, it had an estimated development cost of US$300 million (or about US$1 billion in 2006 constant dollars), but by the time it was sent into orbit in 1990, the cost was about four times that. In addition, new instruments and servicing missions increased the cost to at least US$9 billion by 2006.
Scientific results
The JWST completed its commissioning and began full scientific operations on 11 July 2022. With some exceptions, most experiment data is kept private for one year for the exclusive use of scientists running that particular experiment, and then the raw data is released to the public.
JWST observations substantially advanced understanding of exoplanets, the first billion years of the universe, and other astrophysical and cosmological phenomena.
First full-color images
The first full-color images and spectroscopic data were released on 12 July 2022, which also marked the official beginning of Webb's general science operations. U.S. President Joe Biden revealed the first image, Webb's First Deep Field, on 11 July 2022. Additional releases around this time include:
- Carina Nebula – young, star-forming region called NGC 3324 about 8,500 light-years from Earth, described by NASA as "Cosmic Cliffs".
- WASP-96b – including an analysis of atmosphere with evidence of water around a giant gas planet orbiting a distant star 1120 light-years from Earth.
- Southern Ring Nebula – clouds of gas and dust expelled by a dying star 2,500 light-years from Earth.
- Stephan's Quintet – a visual display of five galaxies with colliding gas and dust clouds creating new stars; four central galaxies are 290 million light-years from Earth.
- SMACS J0723.3-7327 – a galaxy cluster at redshift 0.39, with distant background galaxies whose images are distorted and magnified due to gravitational lensing by the cluster. This image has been called Webb's First Deep Field. It was later discovered that in this picture were three ancient galaxies that existed shortly after the Big Bang. The images of these distant galaxies are views of the universe 13.1 billion years ago.
On 14 July 2022, NASA presented images of Jupiter and related areas by the JWST, including infrared views.
In a preprint released around the same time, NASA, ESA and CSA scientists stated that "the science performance of JWST is better than expected". The document stated that during the commissioning, the instruments captured spectra of transiting exoplanets with a precision better than 1000 ppm per data point, and tracked moving objects with speeds up to 67 milliarcseconds/second, more than twice as fast as the requirement. It also obtained the spectra of hundreds of stars simultaneously in a dense field towards the Milky Way's Galactic Center. Other targets included:
- Moving targets: Jupiter's rings and moons (particularly Europa, Thebe and Metis), asteroids 2516 Roman, 118 Peitho, 6481 Tenzing, 1773 Rumpelstilz, 216 Kleopatra, 2035 Stearns, 4015 Wilson-Harrington and 2004 JX20
- NIRCam grism time-series, NIRISS SOSS and NIRSpec BOTS mode: the Jupiter-sized planet HAT-P-14b
- NIRISS aperture masking interferometry (AMI): A clear detection of the very low-mass companion star AB Doradus C, which had a separation of only 0.3 arcseconds to the primary. This observation was the first demonstration of AMI in space.
- MIRI low-resolution spectroscopy (LRS): a hot super-Earth planet L 168-9 b (TOI-134) around a bright M-dwarf star (red dwarf star)
Bright early galaxies
Within two weeks of the first Webb images, several preprint papers described a wide range of high redshift and very luminous (presumably large) galaxies believed to date from 235 million years (z=16.7) to 280 million years after the Big Bang, far earlier than previously known. On 17 August 2022, NASA released a large mosaic image of 690 individual frames taken by the NIRCam on Webb of numerous very early galaxies. Some early galaxies observed by Webb like CEERS-93316, which has an estimated redshift of approximately z=16.7 corresponding to 235.8 million years after the Big Bang, are high redshift galaxy candidates. In September 2022, primordial black holes were proposed as explaining these unexpectedly large and early galaxies. In May 2024, the JWST identified the most distant known galaxy, JADES-GS-z14-0, seen just 290 million years after the Big Bang, corresponding to a redshift of 14.32. Part of the JWST Advanced Deep Extragalactic Survey (JADES), this discovery highlights a galaxy significantly more luminous and massive than expected for such an early period. Detailed analysis using JWST's NIRSpec and MIRI instruments revealed this galaxy's remarkable properties, including its significant size and dust content, challenging current models of early galaxy formation.
Gallery
- First images by the James Webb Space Telescope – released 12 July 2022
-
Cosmic Cliffs of Carina Nebula (NGC 3324) (NIRCam)
-
Carina Nebula (NGC 3324) (MIRI)
-
Webb's First Deep Field (SMACS 0723; Left: MIRI; Right: NIRCam)
-
Stephan's Quintet (NIRCam/MIRI composite)
Images for kids
-
Alternative Hubble Space Telescope views of the Carina Nebula, comparing ultraviolet and visible (top) and infrared (bottom) astronomy. Far more stars are visible in the latter.
-
JWST as seen from the ESC-D Cryotechnic upper stage shortly after separation, approximately 29 minutes after launch. Part of the Earth with the Gulf of Aden is visible in the background of the image.
-
Segment alignment begins by defocusing the segment images by moving the secondary mirror slightly. Mathematical analysis, called phase retrieval, is applied to the defocused images to determine the precise positioning errors of the segments. Adjustments of the segments then result in 18 well-corrected "telescopes". However, the segments still do not work together as a single mirror.
-
Image stacking. To put all of the light in a single place, each segment image must be stacked on top of one another. In the image stacking step, the individual segment images are moved so that they fall precisely at the center of the field to produce one unified image. This process prepares the telescope for coarse phasing.
-
Image comparison between "old" Spitzer and new JWST
See also
In Spanish: Telescopio espacial James Webb para niños