Extrasolar planet facts for kids
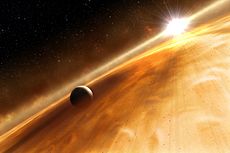
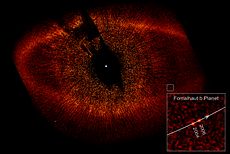
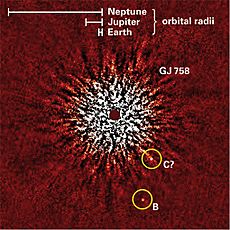
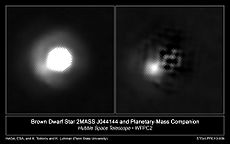
An extrasolar planet (or exoplanet) is a natural planet in a planetary system outside our own solar system.
In 2013, estimates of the number of terrestrial planets in the Milky Way ranged from at least 17 billion to at least 144 billion. The smaller estimate studied planet candidates gathered by the Kepler space observatory. Among them are 461 Earth-size planets, at least four of which are in the "habitable zone" where liquid water can exist. One of the four, dubbed Kepler-69c, is a mere 1.5 times the size of the Earth and around a star like our own Sun – about as near as the current data allow to finding an "Earth 2.0".
Earlier work suggested that there are at least 100 billion planets of all types in our galaxy, an average of at least one per star. There are also planets that orbit brown dwarfs, and free-floating planets that orbit the galaxy directly just as the stars do. It is unclear whether either type should be called a "planet".
Analogies with planets in the Solar System apply to few of the extrasolar planets known. Most are quite unlike any of our planets, for example the so-called "hot Jupiters".
Contents
History
Early speculations
In the sixteenth century, the Italian philosopher Giordano Bruno, an early supporter of the Copernican theory that the Earth and other planets orbit the Sun, put forward the view that the fixed stars are similar to the Sun and are likewise accompanied by planets. Bruno was burnt at the stake by the Holy Inquisition.
In the eighteenth century, the same possibility was mentioned by Isaac Newton in his Principia. Making a comparison to the Sun's planets, he wrote "And if the fixed stars are the centres of similar systems, they will all be constructed according to a similar design and subject to the dominion of One".
Confirmed discoveries
The first published and confirmed discovery was made in 1988. It was finally confirmed in 1992.
In 1992, radio astronomers announced the discovery of planets around another pulsar. These pulsar planets are believed to have formed from the unusual remnants of the supernova that produced the pulsar, in a second round of planet formation. Otherwise they may be the remaining rocky cores of gas giants that survived the supernova and then decayed into their current orbits.
On October 6, 1995, Michel Mayor and Didier Queloz of the University of Geneva announced the first definitive detection of an exoplanet orbiting an ordinary main-sequence star (51 Pegasi). This discovery, made at the Observatoire de Haute-Provence, started the modern era of exoplanetary discovery. Technological advances, most notably in high-resolution spectroscopy, led to the quick detection of many new exoplanets. These advances allowed astronomers to detect exoplanets indirectly by measuring their gravitational influence on the motion of their parent stars. Additional extrasolar planets were eventually detected by watching occultations when a star becomes dimmer as an orbiting planet passed in front of it.
In May 2016 NASA announced the discovery of 1,284 exoplanets which brought the total number of exoplanets to over 3,000.
Formation and evolution
Planets may form within a few to tens (or more) of millions of years of their star forming. The planets of the Solar System can only be observed in their current state, but observations of different planetary systems of varying ages allows us to observe planets at different stages of evolution. Available observations range from young proto-planetary disks where planets are still forming to planetary systems of over 10 Gyr old. When planets form in a gaseous protoplanetary disk, they accrete hydrogen/helium envelopes. These envelopes cool and contract over time and, depending on the mass of the planet, some or all of the hydrogen/helium is eventually lost to space. This means that even terrestrial planets may start off with large radii if they form early enough. An example is Kepler-51b which has only about twice the mass of Earth but is almost the size of Saturn, which is a hundred times the mass of Earth. Kepler-51b is quite young at a few hundred million years old.
Planet-hosting stars
There is at least one planet on average per star. About 1 in 5 Sun-like stars have an "Earth-sized" planet in the habitable zone.
Most known exoplanets orbit stars roughly similar to the Sun, i.e. main-sequence stars of spectral categories F, G, or K. Lower-mass stars (red dwarfs, of spectral category M) are less likely to have planets massive enough to be detected by the radial-velocity method. Despite this, several tens of planets around red dwarfs have been discovered by the Kepler telescope, which uses the transit method to detect smaller planets.
Using data from Kepler, a correlation has been found between the metallicity of a star and the probability that the star hosts a giant planet, similar to the size of Jupiter. Stars with higher metallicity are more likely to have planets, especially giant planets, than stars with lower metallicity.
Some planets orbit one member of a binary star system, and several circumbinary planets have been discovered which orbit both members of a binary star. A few planets in triple star systems are known and one in the quadruple system Kepler-64.
General features
Color and brightness
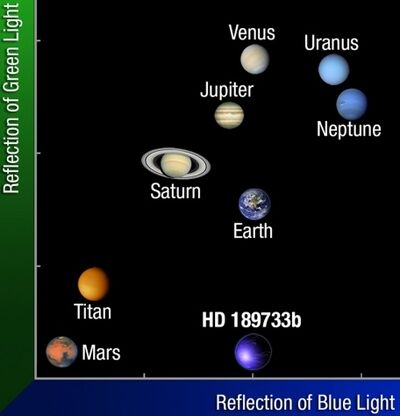
In 2013, the color of an exoplanet was determined for the first time. The best-fit albedo measurements of HD 189733b suggest that it is deep dark blue. Later that same year, the colors of several other exoplanets were determined, including GJ 504 b which visually has a magenta color, and Kappa Andromedae b, which if seen up close would appear reddish in color. Helium planets are expected to be white or grey in appearance.
The apparent brightness (apparent magnitude) of a planet depends on how far away the observer is, how reflective the planet is (albedo), and how much light the planet receives from its star, which depends on how far the planet is from the star and how bright the star is. So, a planet with a low albedo that is close to its star can appear brighter than a planet with a high albedo that is far from the star.
The darkest known planet in terms of geometric albedo is TrES-2b, a hot Jupiter that reflects less than 1% of the light from its star, making it less reflective than coal or black acrylic paint. Hot Jupiters are expected to be quite dark due to sodium and potassium in their atmospheres, but it is not known why TrES-2b is so dark—it could be due to an unknown chemical compound.
For gas giants, geometric albedo generally decreases with increasing metallicity or atmospheric temperature unless there are clouds to modify this effect. Increased cloud-column depth increases the albedo at optical wavelengths, but decreases it at some infrared wavelengths. Optical albedo increases with age, because older planets have higher cloud-column depths. Optical albedo decreases with increasing mass, because higher-mass giant planets have higher surface gravities, which produces lower cloud-column depths. Also, elliptical orbits can cause major fluctuations in atmospheric composition, which can have a significant effect.
There is more thermal emission than reflection at some near-infrared wavelengths for massive and/or young gas giants. So, although optical brightness is fully phase-dependent, this is not always the case in the near infrared.
Temperatures of gas giants reduce over time and with distance from their stars. Lowering the temperature increases optical albedo even without clouds. At a sufficiently low temperature, water clouds form, which further increase optical albedo. At even lower temperatures, ammonia clouds form, resulting in the highest albedos at most optical and near-infrared wavelengths.
Magnetic field
In 2014, a magnetic field around HD 209458 b was inferred from the way hydrogen was evaporating from the planet. It is the first (indirect) detection of a magnetic field on an exoplanet. The magnetic field is estimated to be about one-tenth as strong as Jupiter's.
The magnetic fields of exoplanets may be detectable by their auroral radio emissions with sensitive enough radio telescopes such as LOFAR. The radio emissions could enable determination of the rotation rate of the interior of an exoplanet, and may yield a more accurate way to measure exoplanet rotation than by examining the motion of clouds.
Earth's magnetic field results from its flowing liquid metallic core, but on massive super-Earths with high pressure, different compounds may form which do not match those created under terrestrial conditions. Compounds may form with greater viscosities and high melting temperatures, which could prevent the interiors from separating into different layers and so result in undifferentiated coreless mantles. Forms of magnesium oxide such as MgSi3O12 could be a liquid metal at the pressures and temperatures found in super-Earths and could generate a magnetic field in the mantles of super-Earths.
Hot Jupiters have been observed to have a larger radius than expected. This could be caused by the interaction between the stellar wind and the planet's magnetosphere creating an electric current through the planet that heats it up (Joule heating) causing it to expand. The more magnetically active a star is, the greater the stellar wind and the larger the electric current leading to more heating and expansion of the planet. This theory matches the observation that stellar activity is correlated with inflated planetary radii.
In August 2018, scientists announced the transformation of gaseous deuterium into a liquid metallic hydrogen form. This may help researchers better understand giant gas planets, such as Jupiter, Saturn and related exoplanets, since such planets are thought to contain a lot of liquid metallic hydrogen, which may be responsible for their observed powerful magnetic fields.
Although scientists previously announced that the magnetic fields of close-in exoplanets may cause increased stellar flares and starspots on their host stars, in 2019 this claim was demonstrated to be false in the HD 189733 system. The failure to detect "star-planet interactions" in the well-studied HD 189733 system calls other related claims of the effect into question.
In 2019, the strength of the surface magnetic fields of 4 hot Jupiters were estimated and ranged between 20 and 120 gauss compared to Jupiter's surface magnetic field of 4.3 gauss.
Plate tectonics
In 2007, two independent teams of researchers came to opposing conclusions about the likelihood of plate tectonics on larger super-Earths with one team saying that plate tectonics would be episodic or stagnant and the other team saying that plate tectonics is very likely on super-Earths even if the planet is dry.
If super-Earths have more than 80 times as much water as Earth, then they become ocean planets with all land completely submerged. However, if there is less water than this limit, then the deep water cycle will move enough water between the oceans and mantle to allow continents to exist.
Volcanism
Large surface temperature variations on 55 Cancri e have been attributed to possible volcanic activity releasing large clouds of dust which blanket the planet and block thermal emissions.
Rings
The star 1SWASP J140747.93-394542.6 is orbited by an object that is circled by a ring system much larger than Saturn's rings. However, the mass of the object is not known; it could be a brown dwarf or low-mass star instead of a planet.
The brightness of optical images of Fomalhaut b could be due to starlight reflecting off a circumplanetary ring system with a radius between 20 and 40 times that of Jupiter's radius, about the size of the orbits of the Galilean moons.
The rings of the Solar System's gas giants are aligned with their planet's equator. However, for exoplanets that orbit close to their star, tidal forces from the star would lead to the outermost rings of a planet being aligned with the planet's orbital plane around the star. A planet's innermost rings would still be aligned with the planet's equator so that if the planet has a tilted rotational axis, then the different alignments between the inner and outer rings would create a warped ring system.
Moons
In December 2013 a candidate exomoon of a rogue planet was announced. On 3 October 2018, evidence suggesting a large exomoon orbiting Kepler-1625b was reported.
Atmospheres
Atmospheres have been detected around several exoplanets. The first to be observed was HD 209458 b in 2001.
As of February 2014, more than fifty transiting and five directly imaged exoplanet atmospheres have been observed, resulting in detection of molecular spectral features; observation of day–night temperature gradients; and constraints on vertical atmospheric structure. Also, an atmosphere has been detected on the non-transiting hot Jupiter Tau Boötis b.
In May 2017, glints of light from Earth, seen as twinkling from an orbiting satellite a million miles away, were found to be reflected light from ice crystals in the atmosphere. The technology used to determine this may be useful in studying the atmospheres of distant worlds, including those of exoplanets.
Comet-like tails
KIC 12557548 b is a small rocky planet, very close to its star, that is evaporating and leaving a trailing tail of cloud and dust like a comet. The dust could be ash erupting from volcanos and escaping due to the small planet's low surface-gravity, or it could be from metals that are vaporized by the high temperatures of being so close to the star with the metal vapor then condensing into dust.
In June 2015, scientists reported that the atmosphere of GJ 436 b was evaporating, resulting in a giant cloud around the planet and, due to radiation from the host star, a long trailing tail 14 million km (9 million mi) long.
Insolation pattern
Tidally locked planets in a 1:1 spin-orbit resonance would have their star always shining directly overhead on one spot, which would be hot with the opposite hemisphere receiving no light and being freezing cold. Such a planet could resemble an eyeball, with the hotspot being the pupil. Planets with an eccentric orbit could be locked in other resonances. 3:2 and 5:2 resonances would result in a double-eyeball pattern with hotspots in both eastern and western hemispheres. Planets with both an eccentric orbit and a tilted axis of rotation would have more complicated insolation patterns.
Surface
Surface composition
Surface features can be distinguished from atmospheric features by comparing emission and reflection spectroscopy with transmission spectroscopy. Mid-infrared spectroscopy of exoplanets may detect rocky surfaces, and near-infrared may identify magma oceans or high-temperature lavas, hydrated silicate surfaces and water ice, giving an unambiguous method to distinguish between rocky and gaseous exoplanets.
Surface temperature
Measuring the intensity of the light it receives from its parent star can estimate the temperature of an exoplanet. For example, the planet OGLE-2005-BLG-390Lb is estimated to have a surface temperature of roughly −220 °C (50 K). However, such estimates may be substantially in error because they depend on the planet's usually unknown albedo, and because factors such as the greenhouse effect may introduce unknown complications. A few planets have had their temperature measured by observing the variation in infrared radiation as the planet moves around in its orbit and is eclipsed by its parent star. For example, the planet HD 189733b has been estimated to have an average temperature of 1,205 K (932 °C) on its dayside and 973 K (700 °C) on its nightside.
Habitability
As more planets are discovered, the field of exoplanetology continues to grow into a deeper study of extrasolar worlds, and will ultimately tackle the prospect of life on planets beyond the Solar System. At cosmic distances, life can only be detected if it is developed at a planetary scale and strongly modified the planetary environment, in such a way that the modifications cannot be explained by classical physico-chemical processes (out of equilibrium processes). For example, molecular oxygen (O2) in the atmosphere of Earth is a result of photosynthesis by living plants and many kinds of microorganisms, so it can be used as an indication of life on exoplanets, although small amounts of oxygen could also be produced by non-biological means. Furthermore, a potentially habitable planet must orbit a stable star at a distance within which planetary-mass objects with sufficient atmospheric pressure can support liquid water at their surfaces.
Habitable zone
The habitable zone around a star is the region where the temperature is just right to allow liquid water to exist on the surface of a planet; that is, not too close to the star for the water to evaporate and not too far away from the star for the water to freeze. The heat produced by stars varies depending on the size and age of the star, so that the habitable zone can be at different distances for different stars. Also, the atmospheric conditions on the planet influence the planet's ability to retain heat so that the location of the habitable zone is also specific to each type of planet: desert planets (also known as dry planets), with very little water, will have less water vapor in the atmosphere than Earth and so have a reduced greenhouse effect, meaning that a desert planet could maintain oases of water closer to its star than Earth is to the Sun. The lack of water also means there is less ice to reflect heat into space, so the outer edge of desert-planet habitable zones is further out. Rocky planets with a thick hydrogen atmosphere could maintain surface water much further out than the Earth–Sun distance. Planets with larger mass have wider habitable zones because gravity reduces the water cloud column depth which reduces the greenhouse effect of water vapor, thus moving the inner edge of the habitable zone closer to the star.
Planetary rotation rate is one of the major factors determining the circulation of the atmosphere and hence the pattern of clouds: slowly rotating planets create thick clouds that reflect more and so can be habitable much closer to their star. Earth with its current atmosphere would be habitable in Venus's orbit, if it had Venus's slow rotation. If Venus lost its water ocean due to a runaway greenhouse effect, it is likely to have had a higher rotation rate in the past. Alternatively, Venus never had an ocean because water vapor was lost to space during its formation and could have had its slow rotation throughout its history.
Tidally locked planets (a.k.a. "eyeball" planets) can be habitable closer to their star than previously thought due to the effect of clouds: at high stellar flux, strong convection produces thick water clouds near the substellar point that greatly increase the planetary albedo and reduce surface temperatures.
Planets in the habitable zones of stars with low metallicity are more habitable for complex life on land than high metallicity stars because the stellar spectrum of high metallicity stars is less likely to cause the formation of ozone thus enabling more ultraviolet rays to reach the planet's surface.
Habitable zones have usually been defined in terms of surface temperature, however over half of Earth's biomass is from subsurface microbes, and the temperature increases with depth, so the subsurface can be conducive for microbial life when the surface is frozen and if this is considered, the habitable zone extends much further from the star, even rogue planets could have liquid water at sufficient depths underground. In an earlier era of the universe the temperature of the cosmic microwave background would have allowed any rocky planets that existed to have liquid water on their surface regardless of their distance from a star. Jupiter-like planets might not be habitable, but they could have habitable moons.
Ice ages and snowball states
The outer edge of the habitable zone is where planets are completely frozen, but planets well inside the habitable zone can periodically become frozen. If orbital fluctuations or other causes produce cooling, then this creates more ice, but ice reflects sunlight causing even more cooling, creating a feedback loop until the planet is completely or nearly completely frozen. When the surface is frozen, this stops carbon dioxide weathering, resulting in a build-up of carbon dioxide in the atmosphere from volcanic emissions. This creates a greenhouse effect which thaws the planet again. Planets with a large axial tilt are less likely to enter snowball states and can retain liquid water further from their star. Large fluctuations of axial tilt can have even more of a warming effect than a fixed large tilt. Paradoxically, planets orbiting cooler stars, such as red dwarfs, are less likely to enter snowball states because the infrared radiation emitted by cooler stars is mostly at wavelengths that are absorbed by ice which heats it up.
Tidal heating
If a planet has an eccentric orbit, then tidal heating can provide another source of energy besides stellar radiation. This means that eccentric planets in the radiative habitable zone can be too hot for liquid water. Tides also circularize orbits over time, so there could be planets in the habitable zone with circular orbits that have no water because they used to have eccentric orbits. Eccentric planets further out than the habitable zone would still have frozen surfaces, but the tidal heating could create a subsurface ocean similar to Europa's. In some planetary systems, such as in the Upsilon Andromedae system, the eccentricity of orbits is maintained or even periodically varied by perturbations from other planets in the system. Tidal heating can cause outgassing from the mantle, contributing to the formation and replenishment of an atmosphere.
Types
Extrasolar planets can have many different forms.
- They can be gas giants or rocky planets
- They can possibly be dwarf planets, i.e. planets smaller and less dense than regular planets
- They can orbit several different types of stars
- They can be free-floating or orbit a brown dwarf
- They may support life. One recently discovered exoplanet, Gliese 581g is thought to possibly support life, but the existence of this planet is not yet confirmed.
Nearest
The nearest star with planets is Alpha Centauri. It is 4.3 light years away. Using standard rockets, it would take tens of thousands of years to get there. The nearest star similar to our Sun is Tau Ceti. It has five planets, one of which in the habitable zone, where liquid water may exist.
Most Earth-like
Some extrasolar planets might be Earth-like. This means that they have conditions very similar to that of the Earth. Planets are ranked by a formula called the Earth similarity index or ESI for short. The ESI goes from one (most Earth-like) to zero (least Earth-like). For a planet to be habitable it should have an ESI of at least 0.8. For comparison, the four solar terrestrial planets are included in this list.
Name | ESI | SFV | HZD | COM | ATM | Planet type | Star | Habitability | Distance (ly) | Status | Year of discovery |
Ref |
---|---|---|---|---|---|---|---|---|---|---|---|---|
Earth | 1.00 | 0.72 | −0.50 | −0.31 | −0.52 | warm terran | G | mesoplanet | 0 | Non-exoplanet, inhabited | prehistoric | |
Kepler-438b | 0.88 | 0.88 | −0.93 | −0.14 | −0.73 | warm terran | M | mesoplanet | 472.9 | confirmed | 2015 | |
Kepler-1410b | 0.88 | 0.63 | −0.88 | −0.16 | −0.06 | warm superterran | K | mesoplanet | 1213.4 | confirmed | 2011 | |
Gliese 667 Cc | 0.84 | 0.64 | −0.62 | −0.15 | +0.21 | warm terran | M | mesoplanet | 23.6 | confirmed | 2011 | |
Kepler-442b | 0.83 | 0.98 | −0.72 | −0.15 | +0.28 | warm superterran | K | mesoplanet | 1291.6 | confirmed | 2015 | |
Kepler-62e | 0.83 | 0.96 | −0.70 | −0.15 | +0.28 | warm superterran | K | mesoplanet | 1199.7 | confirmed | 2013 | |
Kepler-452b | 0.83 | 0.93 | −0.61 | -0.15 | -0.30 | warm superterran | G | mesoplanet | 1402.5 | confirmed | 2015 | |
Gliese 832 c | 0.81 | 0.96 | −0.72 | −0.15 | +0.43 | warm superterran | M | mesoplanet | 16.1 | confirmed | 2014 | |
Kepler-283c | 0.79 | 0.85 | −0.58 | −0.14 | +0.69 | warm superterran | K | mesoplanet | 1496.8 | confirmed | 2011 | |
Kepler-436b | 0.79 | 0.33 | −0.87 | −0.14 | +0.47 | warm superterran | M | mesoplanet | 1339.4 | confirmed | 2015 | |
Kepler-1229b | 0.79 | 0.00 | −0.40 | −0.15 | +0.44 | warm superterran | M | mesoplanet | 769.7 | confirmed | 2016 | |
Tau Ceti e | 0.78 | 0.00 | −0.92 | −0.15 | +0.16 | warm superterran | G | mesoplanet | 11.9 | unconfirmed | 2012 | |
Kepler-296f | 0.78 | 0.15 | −0.90 | −0.14 | +0.53 | warm superterran | M | mesoplanet | 1089.6 | confirmed | 2011 | |
Gliese 180 c | 0.77 | 0.42 | −0.53 | −0.14 | +0.64 | warm superterran | M | mesoplanet | 39.5 | unconfirmed | 2014 | |
Gliese 667 Cf | 0.77 | 0.00 | -0.22 | −0.16 | +0.08 | warm terran | M | psychroplanet | 23.6 | dubious | 2013 | |
Gliese 581 g | 0.76 | 1.00 | -0.70 | −0.15 | +0.28 | warm superterran | M | mesoplanet | 20.2 | dubious | 2010 | |
Gliese 163 c | 0.75 | 0.02 | −0.96 | −0.14 | +0.58 | warm superterran | M | mesoplanet | 48.9 | confirmed | 2012 | |
Gliese 180 b | 0.75 | 0.41 | −0.88 | −0.14 | +0.74 | warm superterran | M | mesoplanet | 39.5 | unconfirmed | 2014 | |
HD 40307 g | 0.74 | 0.04 | −0.23 | −0.14 | +0.77 | warm superterran | K | psychroplanet | 41.7 | confirmed | 2012 | |
Kepler-61b | 0.73 | 0.27 | −0.88 | −0.13 | +1.24 | warm superterran | M | mesoplanet | 1062.8 | confirmed | 2013 | |
Kepler-443b | 0.73 | 0.91 | −0.49 | −0.13 | +1.44 | warm superterran | K | mesoplanet | 2564.4 | confirmed | 2015 | |
Gliese 422 b | 0.71 | 0.17 | −0.41 | −0.13 | +1.11 | warm megaterran | M | mesoplanet | 41.3 | unconfirmed | 2014 | |
Kepler-22b | 0.71 | 0.53 | −0.64 | −0.12 | +1.79 | warm superterran | G | mesoplanet | 619.4 | confirmed | 2011 | |
Kepler-440b | 0.70 | 0.00 | +0.01 | −0.15 | +0.38 | warm superterran | K | psychroplanet | 706.5 | confirmed | 2015 | |
Kepler-298d | 0.68 | 0.00 | −0.86 | −0.11 | +2.11 | warm superterran | K | mesoplanet | 1545 | confirmed | 2012 | |
Kepler-439b | 0.68 | 0.00 | −0.99 | −0.13 | +1.18 | warm superterran | G | thermoplanet | 1914.8 | confirmed | 2015 | |
Kapteyn b | 0.67 | 0.00 | +0.08 | −0.15 | +0.57 | warm superterran | M | psychroplanet | 12.7 | unconfirmed | 2014 | |
Kepler-62f | 0.67 | 0.05 | +0.45 | −0.16 | +0.19 | warm superterran | K | psychroplanet | 1199.7 | confirmed | 2013 | |
Kepler-186f | 0.64 | 0.00 | +0.48 | −0.17 | −0.26 | warm terran | M | psychroplanet | 492 | confirmed | 2014 | |
Kepler-174d | 0.61 | 0.00 | +0.32 | −0.13 | +1.77 | warm superterran | K | psychroplanet | 878.3 | confirmed | 2011 | |
Gliese 667 Ce | 0.60 | 0.00 | +0.51 | −0.16 | +0.23 | warm terran | M | psychroplanet | 23.6 | dubious | 2013 | |
Gliese 682 c | 0.59 | 0.00 | +0.22 | −0.14 | +1.19 | warm superterran | M | psychroplanet | 16.6 | unconfirmed | 2014 | |
Gliese 581 d | 0.53 | 0.00 | +0.78 | −0.14 | +0.94 | warm superterran | M | hypopsychroplanet | 20.2 | unconfirmed | 2007 | |
Kepler-155c | K | - | confirmed | |||||||||
Venus | 0.78 | 0.00 | −0.93 | −0.28 | −0.70 | warm terran | G | hyperthermoplanet | close to zero | non-exoplanet | prehistoric | |
Mars | 0.64 | 0.00 | +0.33 | −0.13 | −1.12 | warm subterran | G | hypopsychroplanet | close to zero | non-exoplanet | prehistoric | |
Mercury | 0.39 | 0.00 | −1.46 | −0.52 | −1.37 | hot mercurian | G | non-habitable | close to zero | non-exoplanet | prehistoric |
Potentially habitable planets
A review in 2015 identified exoplanets Kepler-62f, Kepler-186f and Kepler-442b as the best candidates for being potentially habitable. These are at a distance of 1200, 490 and 1,120 light-years away, respectively. Of these, Kepler-186f is in similar size to Earth with its 1.2-Earth-radius measure, and it is located towards the outer edge of the habitable zone around its red dwarf star.
When looking at the nearest terrestrial exoplanet candidates, Proxima Centauri b is about 4.2 light-years away. Its equilibrium temperature is estimated to be −39 °C (234 K).
Earth-size planets
- In November 2013, it was estimated that 22±8% of Sun-like stars in the Milky Way galaxy may have an Earth-sized planet in the habitable zone. Assuming 200 billion stars in the Milky Way, that would be 11 billion potentially habitable Earths, rising to 40 billion if red dwarfs are included.
- Kepler-186f, a 1.2-Earth-radius planet in the habitable zone of a red dwarf, was reported in April 2014.
- Proxima Centauri b, a planet in the habitable zone of Proxima Centauri, the nearest known star to the solar system with an estimated minimum mass of 1.27 times the mass of the Earth.
- In February 2013, researchers speculated that up to 6% of small red dwarfs may have Earth-size planets. This suggests that the closest one to the Solar System could be 13 light-years away. The estimated distance increases to 21 light-years when a 95% confidence interval is used. In March 2013, a revised estimate gave an occurrence rate of 50% for Earth-size planets in the habitable zone of red dwarfs.
- At 1.63 times Earth's radius Kepler-452b is the first discovered near-Earth-size planet in the "habitable zone" around a G2-type Sun-like star (July 2015).
Related pages
Images for kids
-
Coronagraphic image of AB Pictoris showing a companion (bottom left), which is either a brown dwarf or a massive planet. The data was obtained on 16 March 2003 with NACO on the VLT, using a 1.4 arcsec occulting mask on top of AB Pictoris.
See also
In Spanish: Planeta extrasolar para niños