Star facts for kids
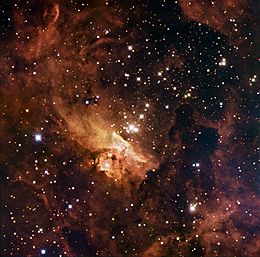
The strange shapes taken by the clouds are a result of the vast radiation emitted by these huge, hot stars.
This image combines image data with three different filters in visible light from the 1.5-metre Danish telescope at the ESO La Silla Observatory in Chile.
A star is a massive ball of plasma (very hot gas) held together by gravity. It radiates energy because of the nuclear reactions inside it
It radiates heat and light, and every other part of the electromagnetic spectrum, such as radio waves, micro-waves, X-rays, gamma-rays and ultra-violet radiation. The proportions vary according to the mass and age of the star.
The energy of stars comes from nuclear fusion. This is a process that turns a light chemical element into another heavier element. Stars are mostly made of hydrogen and helium. They turn the hydrogen into helium by fusion. When a star is near the end of its life, it begins to change the helium into other heavier chemical elements, like carbon and oxygen. Fusion produces a lot of energy. The energy makes the star very hot. The energy produced by stars radiates away from them. The energy leaves as electromagnetic radiation.
Contents
Birth of a star
A star begins as a collapsing cloud of material made mostly of hydrogen, with helium and tiny amounts of heavier elements. Once the stellar core is dense enough, some of the hydrogen is changed into helium through nuclear fusion. The energy moves away from the core by a combination of radiation and convection. Early stars of less than 2 M☉ are called T Tauri stars, while those with greater mass are Herbig Ae/Be stars.
Main sequence
Stars spend about 90% of their lifetimes fusing hydrogen into helium in high-temperature-and-pressure reactions in their cores. Such stars are said to be on the main sequence and are called dwarf stars.
Starting at zero-age main sequence, the proportion of helium in a star's core will steadily increase, the rate of nuclear fusion at the core will slowly increase, as will the star's temperature and luminosity.
The Sun, for example, is estimated to have increased in luminosity by about 40% since it reached the main sequence 4.6 billion (4.6×109) years ago.
Every star generates a stellar wind of particles that causes a continual outflow of gas into space. For most stars, the mass lost is negligible. The Sun loses 10−14 M☉ every year, or about 0.01% of its total mass over its entire lifespan. However, very massive stars can lose 10−7 to 10−5 M☉ each year, significantly affecting their evolution. Stars that begin with more than 50 M☉ can lose over half their total mass while on the main sequence.
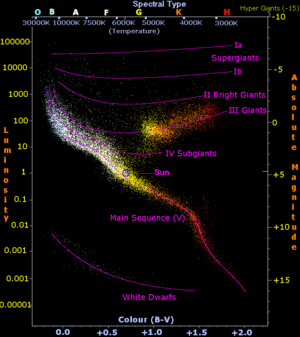
The time a star spends on the main sequence depends primarily on the amount of fuel it has and the rate at which it fuses it. The Sun is expected to live 10 billion (1010) years. Massive stars consume their fuel very rapidly and are short-lived. Low mass stars consume their fuel very slowly. Stars less massive than 0.25 M☉, called red dwarfs, are able to fuse nearly all of their mass while stars of about 1 M☉ can only fuse about 10% of their mass. The combination of their slow fuel-consumption and relatively large usable fuel supply allows low mass stars to last about one trillion (10×1012) years; the most extreme of 0.08 M☉ will last for about 12 trillion years.
Red dwarfs become hotter and more luminous as they accumulate helium. When they eventually run out of hydrogen, they contract into a white dwarf and decline in temperature. Since the lifespan of such stars is greater than the current age of the universe (13.8 billion years), no stars under about 0.85 M☉ are expected to have moved off the main sequence.
Besides mass, the elements heavier than helium can play a significant role in the evolution of stars. Astronomers label all elements heavier than helium "metals", and call the chemical concentration of these elements in a star, its metallicity. A star's metallicity can influence the time the star takes to burn its fuel, and controls the formation of its magnetic fields, which affects the strength of its stellar wind. Older, population II stars have substantially less metallicity than the younger, population I stars due to the composition of the molecular clouds from which they formed. Over time, such clouds become increasingly enriched in heavier elements as older stars die and shed portions of their atmospheres.
Post–main sequence
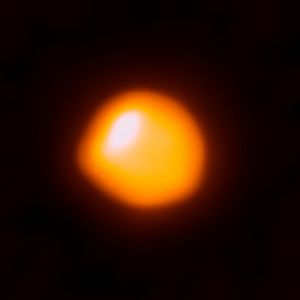
As stars of at least 0.4 M☉ exhaust the supply of hydrogen at their core, they start to fuse hydrogen in a shell surrounding the helium core. The outer layers of the star expand and cool greatly as they transition into a red giant. In some cases, they will fuse heavier elements at the core or in shells around the core. As the stars expand, they throw part of their mass, enriched with those heavier elements, into the interstellar environment, to be recycled later as new stars. In about 5 billion years, when the Sun enters the helium burning phase, it will expand to a maximum radius of roughly 1 astronomical unit (150 million kilometres), 250 times its present size, and lose 30% of its current mass.
As the hydrogen-burning shell produces more helium, the core increases in mass and temperature. In a red giant of up to 2.25 M☉, the mass of the helium core becomes degenerate prior to helium fusion. Finally, when the temperature increases sufficiently, core helium fusion begins explosively in what is called a helium flash, and the star rapidly shrinks in radius and increases its surface temperature. For more massive stars, helium core fusion starts before the core becomes degenerate, and the star spends some time in the red clump, slowly burning helium, before the outer convective envelope collapses and the star then moves to the horizontal branch.
After a star has fused the helium of its core, it begins fusing helium along a shell surrounding the hot carbon core. The star then follows an evolutionary path called the asymptotic giant branch (AGB) that parallels the other described red-giant phase, but with a higher luminosity. The more massive AGB stars may undergo a brief period of carbon fusion before the core becomes degenerate.
During the AGB phase, the luminosity of the star varies and matter is ejected from the star's atmosphere, ultimately forming a planetary nebula. As much as 50 to 70% of a star's mass can be ejected in this mass loss process. Because energy transport in an AGB star is primarily by convection, this ejected material is enriched with the fusion products dredged up from the core. Therefore, the planetary nebula is enriched with elements like carbon and oxygen. Ultimately, the planetary nebula disperses, enriching the general interstellar medium. Therefore, future generations of stars are made of the "star stuff" from past stars.
Massive stars
During their helium-burning phase, a star of more than 9 solar masses expands to form first a blue and then a red supergiant. Particularly massive stars may evolve to a Wolf–Rayet star.
When helium is exhausted at the core of a massive star, the core contracts and the temperature and pressure rises enough to fuse carbon (see Carbon-burning process). This process continues, with the successive stages being fueled by neon (see neon-burning process), oxygen (see oxygen-burning process), and silicon (see silicon-burning process). Near the end of the star's life, fusion continues along a series of onion-layer shells within a massive star. Each shell fuses a different element, with the outermost shell fusing hydrogen; the next shell fusing helium, and so forth.
The final stage occurs when a massive star begins producing iron. Since iron nuclei are more tightly bound than any heavier nuclei, any fusion beyond iron does not produce a net release of energy.
Collapse
As a star's core shrinks, the intensity of radiation from that surface increases, creating such radiation pressure on the outer shell of gas that it will push those layers away, forming a planetary nebula. If what remains after the outer atmosphere has been shed is less than roughly 1.4 M☉, it shrinks to a relatively tiny object about the size of Earth, known as a white dwarf. White dwarfs lack the mass for further gravitational compression to take place. The electron-degenerate matter inside a white dwarf is no longer a plasma. Eventually, white dwarfs fade into black dwarfs over a very long period of time.
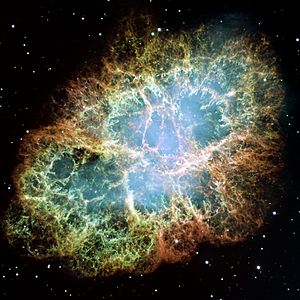
In massive stars, fusion continues until the iron core has grown so large (more than 1.4 M☉) that it can no longer support its own mass. This core will suddenly collapse as its electrons are driven into its protons, forming neutrons, neutrinos, and gamma rays in a burst of electron capture and inverse beta decay. The shockwave formed by this sudden collapse causes the rest of the star to explode in a supernova. Supernovae become so bright that they may briefly outshine the star's entire home galaxy. When they occur within the Milky Way, supernovae have historically been observed by naked-eye observers as "new stars" where none seemingly existed before.
A supernova explosion blows away the star's outer layers, leaving a remnant such as the Crab Nebula. The core is compressed into a neutron star, which sometimes manifests itself as a pulsar or X-ray burster. In the case of the largest stars, the remnant is a black hole greater than 4 M☉.
The blown-off outer layers of dying stars include heavy elements, which may be recycled during the formation of new stars. These heavy elements allow the formation of rocky planets. The outflow from supernovae and the stellar wind of large stars play an important part in shaping the interstellar medium.
Earth and Sun
The star nearest to Earth is the Sun. It is not accurate to say "most of the energy on Earth comes from it". It is more accurate to say the energy of sunlight supports almost all life on Earth by photosynthesis, and drives Earth's climate and weather. The Earth has its own source of heat in its interior: see Age of the Earth. The internal heat comes from the original gravitational formation of the Earth, and from the radioactive materials inside it.
We can see other stars in the night sky when the Sun goes down. They are made mostly of hydrogen and a little bit of helium plus other elements. The sun is such a star.
Planets
Most stars look like shiny dots from Earth, because they are far away. Our Sun is the closest star to us. The earth and other planets move around (orbit) the Sun. The Sun and all things that orbit it are called the Solar System. Many other stars have planets orbiting them: they are called exoplanets.
Numbers, distances
The nearest star to our Solar System, and the second nearest star to Earth after the Sun, is Proxima Centauri. It is 39.9 trillion kilometres away. This is 4.2 light years away, meaning that light from Proxima Centauri takes 4.2 years to reach Earth.
Astronomers think there are a very large number of stars in the Universe. They estimate (guess) that there are at least 70 sextillion stars. That is 70,000,000,000,000,000,000,000; which is about 230 billion times the number of stars in the Milky Way (our galaxy).
Most stars are very old. They are usually thought to be between 1 and 10 billion years old. The oldest stars are thought to be around 13.7 billion years old. Scientists think that is close to the age of the Universe.
Stars vary greatly in size. The smallest neutron stars (which are actually dead stars) are no bigger than a city. The neutron star is incredibly dense. If you were to take a layer a micrometre thick and apply it onto a tank, it would be a very tough armor. The tank would be so heavy, it would sink into the center of the Earth.
Hypergiant stars are the largest stars in the Universe. They have a diameter over 1,500 times bigger than the Sun. If you changed the sun into one of these huge stars down where the sun is, its outer surface would reach beyond the orbit of Jupiter, and the earth would be well inside the star. The star Betelgeuse, in the Orion constellation is a red supergiant star.
When seen in the night sky without a telescope, some stars appear brighter than other stars. This difference is measured in terms of apparent magnitude. There are two reasons for stars to differ in apparent magnitude. If one star is much closer than another otherwise similar star, it will appear much brighter, in just the same way that a candle that is near looks brighter than a big fire that is far away. If one star is much larger or much hotter than another star at about the same distance, it will appear much brighter, in just the same way that if two fires are the same distance away, the bigger or hotter one will look brighter. A star's true luminosity is its absolute magnitude.
Beside light, stars also give off a solar wind and neutrinos. These are very small particles.
Stars are a source of a gravity field. This is what keeps planets close to them. It is also not unusual for two stars to orbit each other. This happens when they are close together. This is also because of gravity, in the same way as the Earth orbits the Sun. These binary stars (binary meaning "two") are thought to be very common. There are even groups of three or more stars orbiting each other. Proxima Centauri is the smallest star in a group of three.
Stars are not spread evenly across all of space. They are grouped into galaxies. A typical galaxy contains hundreds of billions of stars.
History of seeing stars
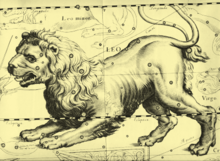
Stars have been important to people all over the world for all of history. Stars have been part of religious practices. Long ago, people believed that stars could never die. Astronomers organized stars into groups called constellations. They used the constellations to help them see the motion of the planets and to guess the position of the Sun. The motion of the Sun and the stars was used to make calendars. The calendars were used by farmers to decide when to plant crops and when to harvest them.
The life of stars
Stars are made in nebulas. These are areas that have more gas than normal space. The gas in a nebula is collected by gravity. The Orion nebula is an example of a place where gas is coming together to form stars.
Stars spend most of their lives burning (fusing) hydrogen to make energy. When hydrogen is fused it makes helium. To fuse hydrogen it must be very hot and the pressure must be very high. Fusion happens at the center of stars, called "the core".
The smallest stars (red dwarfs) fuse their hydrogen slowly and live for 100 billion years. Red dwarfs live longer than any other type of star. At the end of their lives, they become dimmer and dimmer. Red dwarfs do not explode.
When very heavy stars die, they explode. This is called a supernova. When a supernova happens in a nebula, the explosion pushes the gas in the nebula together. This makes the gas in the nebula very thick. Gravity and exploding stars both help to make new stars in nebulas.
Most stars use up the hydrogen at their core. When they do, their core shrinks and becomes very hot. It becomes so hot it pushes away the outer layer of the star. As the outer layer expands it makes a red giant star. Astro-physicists think that in about 5 billion years, the sun will be a red giant. Our sun will be so large it will eat the Earth. After our sun stops using hydrogen to make energy, it will use helium in its very hot core. It will be hotter than when it was fusing hydrogen. Heavy stars will also make elements heavier than helium. As a star makes heavier and heavier elements, it makes less and less energy. Elements as heavy as iron are made in heavy stars.
Average stars (like our sun) will push away their outer gases. The gas it pushes away makes a cloud called a planetary nebula. The core part of the star will remain. It will be a ball as big as the Earth and called a white dwarf. It will fade into a black dwarf over a very long time.
Later in large stars, heavier elements are made by fusion. Finally the star makes a supernova explosion. Most things happen in the universe so slowly we do not notice. But supernova explosions happen in only 100 seconds. When a supernovae explodes its flash is as bright as a 100 billion stars. The dying star is so bright it can be seen during the day. Supernova means "new star" because people used to think it was the beginning of a new star. Today we know that a supernova is the death of an old star. The gas of the star is pushed away by the explosion. It forms a giant cloud of gas called a planetary nebula. The crab nebula is a good example. All that remains is a neutron star. If the star was very heavy, the star will make a black hole. Gravity in a black hole is extremely strong. It is so strong that even light cannot escape from a black hole.
The heaviest elements are made in the explosion of a supernova. After billions of years of floating in space, the gas and dust come together to make new stars and new planets. Much of the gas and dust in space comes from supernovae. Our Sun, the Earth, and all living things are made from star dust.
Colors
Astronomers have known for centuries that stars have different colors. When looking at an electromagnetic spectrum, ultraviolet waves are the shortest, and infrared are the longest. The visible spectrum has wavelengths between these two extremes.
Modern instruments can measure very precisely the color of a star. This allows astronomers to determine that star's temperature, because a hotter star's black-body radiation has shorter wavelengths. The hottest stars are blue and violet, then white, then yellow, and the coolest are red. Knowing the color and absolute magnitude, astronomers can place the star on the Hertzsprung-Russell diagram, and estimate its habitable zone and other facts about it.
For example, our Sun is white, and the Earth is the perfect distance away for life. If our Sun was a hotter, blue star, however, Earth would have to be much farther away or else it would be too hot to have water and sustain life.
Images for kids
-
Infrared image from NASA's Spitzer Space Telescope showing hundreds of thousands of stars in the Milky Way galaxy
-
An example of a Hertzsprung–Russell diagram for a set of stars that includes the Sun (center) (see Classification)
-
Betelgeuse as seen by ALMA. This is the first time that ALMA has observed the surface of a star and resulted in the highest-resolution image of Betelgeuse available.
-
Artist's impression of the Sirius system, a white dwarf star in orbit around an A-type main-sequence star
-
This view of NGC 6397 includes stars known as blue stragglers for their location on the Hertzsprung–Russell diagram.
-
The Pleiades, an open cluster of stars in the constellation of Taurus. These stars share a common motion through space.
-
The reflection nebula NGC 1999 is brilliantly illuminated by V380 Orionis. The black patch of sky is a vast hole of empty space and not a dark nebula as previously thought.
-
The asymmetrical appearance of Mira, an oscillating variable star
-
A cross-section of the Sun
See also
In Spanish: Estrella para niños